陈焕鹏1,2,黄朝峰2 #
(1 广州医科大学附属第三医院生物样本库 广州 510150
2中山大学中山医学院人类病毒学研究所,广州 510080)
作者简介:陈焕鹏 男 博士后 地址:广州市荔湾区多宝路63号 电话:15902075442 邮箱:chhuanp@mail2.sysu.edu.cn
通讯作者:黄朝峰 男 副教授 博士生导师 地址:广州市中山二路74号10号楼N1311室,邮编:510080,电话:8620-87335529,传真:8620-87332588,邮箱:hzhaof@mail.sysu.edu.cn
摘要:嵌合抗原受体(Chimeric antigen receptor,CAR)T细胞是肿瘤免疫治疗领域的新兴疗法,通过基因工程技术改造T细胞,使得CAR T细胞能够结合肿瘤细胞表面的特异性抗原,促使T细胞活化,发挥特异性抗肿瘤作用。嵌合抗原受体结构中的胞内信号区段激活决定了CAR T细胞的增殖分化能力,调控其细胞代谢特征和免疫持久性,进而影响CAR T细胞的临床疗效。本文从不同胞内共刺激信号通路对CAR T细胞的影响和在临床治疗中的差异,分析不同共刺激信号通路在CAR T细胞的受体设计和临床治疗策略选择方面的应用潜力,为CAR T细胞在肿瘤免疫治疗中的精准应用提供理论依据。
关键词:嵌合抗原受体T细胞;肿瘤;共刺激分子
Abstract: Chimeric Antigen Receptor (CAR) T cells is an emerging therapy in tumor immunotherapy. CAR T cells are modified through genetic engineering technology. CAR T cells can bind to the specific antigen on tumor cells to promote T cell full activation and exert anti-tumor activity. The activation of the CAR intracellular signal domain determines the proliferation and differentiation of CAR T cells and then affects the clinical efficacy of CAR T cells. This article will discuss the potential application value of each costimulator from the impact of different costimulatory intracellular signals on CAR T cells and provide a theoretical basis for the precise application of CAR T cells in tumor immunotherapy.
Keywords:CAR T, tumor, costimulator
T细胞活化需要两种信号同时传导,一个是通过T细胞受体(T cell receptor,TCR)与主要组织相容性复合体(MHC)的抗原肽复合物结合介导的第一信号,决定了T细胞应答的特异性;第二个就是T细胞表面的共刺激信号,对于T细胞的完全活化是必要条件。共刺激信号激活可防止T细胞的失能,增强T细胞的活化。嵌合抗原受体(Chimeric antigen receptor,CAR)是一种人工T细胞受体1,与TCR的激活方式不同,CAR能够不依赖于MHC分子的限制性直接识别肿瘤表面抗原,进而活化T细胞2。CAR的结构包括胞外抗原结合结构域的单链抗体(single Chain Fragment Variable,scFv)、间隔域、跨膜结构域和胞内信号传导域组成(图Ⅰ)。缺少了共刺激分子胞内信号的第一代CAR,胞内只含TCR信号中CD3ζ信号传导,致使T细胞无法完全活化,在治疗中没有取得很好的疗效3。而第二代CAR是在一代的基础上融合了共刺激分子的胞内信号与CD3ζ胞内信号4 5,胞内共刺激信号的存在使得CAR T细胞得以被完全的活化,并激活CAR T细胞释放细胞因子,执行细胞毒性功能裂解肿瘤细胞,获得比较好的治疗效果6。共刺激分子主要包括CD28、ICOS、4-1BB、CD27和OX407 8,可增强T细胞增殖和分化,但不同共刺激分子胞内信号对CAR T细胞的活化作用并不完全相同。本文分别讨论不同共刺激分子调控T细胞的作用和融合不同共刺激分子胞内信号对CAR T细胞的影响,总结CAR结构设计对CAR T细胞抗肿瘤作用差异的最新研究进展,为CAR T细胞在肿瘤免疫治疗中的精准应用提供理论依据。
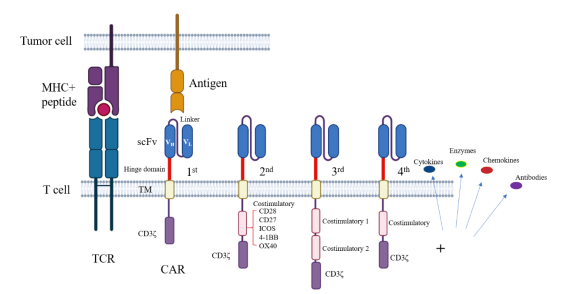
图Ⅰ:TCR与CAR的结构
CD28 CAR
CD28是T淋巴细胞表面主要的共刺激分子,CD28对T细胞活化过程中起关键作用9。CD28的受体为抗原提呈细胞表面的CD80和CD86。CD28的激活会放大TCR信号周期,激活磷酸肌醇3-激酶(PI3K)和蛋白激酶AKT通路,从而激活T细胞的增殖、分化以及效应因子产生10。CD28胞内信号激活YMNM基序内的天冬酰胺募集生长因子受体结合蛋白2(GRB2),并激活NF-AT的活性,诱导T细胞产生更多的IL-211。CD28的激活还上调了葡萄糖转运蛋白1(GLUT1)的表达12,从而增强T细胞的糖酵解途径13。同时,CD28激活还调控了T细胞的线粒体功能14。CD28通过mTOR信号通路调节T细胞的各种代谢途径,包括脂质和糖类的分解代谢15。
CD28作为T细胞激活的最主要的共刺激分子,将CD28胞内结构域融合到CAR中,可以显著的激活CAR T细胞,诱导CAR T细胞的信号转导。CD28胞内信号激活CAR T细胞的糖酵解功能,会诱导产生效应记忆CD8+ T细胞13。最新研究发现,将CAR 结构中CD28胞内信号YMNM基序单个氨基酸残基突变可以促进CAR T细胞的存活和抗肿瘤活性16。融合CD28胞内信号的CAR T细胞表现出迅速的增殖和杀伤肿瘤细胞。但临床结果发现,相比于4-1BB胞内信号的作用,以共刺激分子CD28胞内信号激活的CD19-CAR T细胞在回输治疗后容易引起患者发生脑水肿死亡17,但这是否与CD28胞内信号对CAR T细胞的过度激活有关还需进一步研究证实。
ICOS CAR
诱导型共刺激物(ICOS)也属于CD28超家族成员,是在激活的T细胞上高度表达的共刺激分子18。在TCR和CD28共同刺激后,ICOS被迅速诱导表达19。ICOS和CD28都是CD28家族的成员,可协同调节T细胞分化,功能上具有相似性。ICOS也是通过激活PI3K-AKT信号通路,诱导T细胞的增殖和效应因子的表达20。但ICOS与CD28主要调控的细胞种类有差异。ICOS主要参与调控CD4+ T细胞中T滤泡 辅助细胞(Tfh)和T辅助细胞17(Th17)的细胞分化和扩增21。同时,激活ICOS也被证明可以增强CD8+ T细胞介导的抗肿瘤活性22。ICOS与CD28的不同还在于ICOS的胞内信号区段无法募集GRB2,因此不能诱导T细胞产生IL-223。与CD28一样,ICOS可通过mTOR激活促进T细胞的葡萄糖摄取,调节T细胞的代谢功能24。
融合共刺激分子ICOS胞内信号的CAR T细胞具有更高的PI3K激活和IFN-γ产生,并且ICOS胞内信号增强CAR T细胞的免疫持久性25。ICOS胞内信号激活促进CD4+ CAR T细胞上调表达白介素-17(IL-17)26。ICOS胞内信号传导也诱导CAR T细胞核转录因子T-bet的活性,而T-bet调控了CD4+ T细胞向T辅助细胞1(Th1)细胞的分化。ICOS胞内信号的激活会诱导CAR T细胞向Th17 / Th1细胞分化,而Th17 CAR T会介导高效且持久的抗肿瘤反应26,说明CAR结构中融合ICOS胞内信号主要作用于CD4+ T细胞。CAR的共刺激区段同时包含ICOS胞内信号与4-1BB胞内信号时,可同时增加CD4+ CAR T和CD8+ CAR T细胞抗肿瘤的持久性,包含ICOS胞内信号可显著增强CAR T细胞的功能25。尽管在二代CAR研究中,ICOS构建的CAR T细胞的体内抗肿瘤效果没有优于CD28和4-1BB的CAR。但ICOS调控了CD4+ CAR T细胞的分化和存活,这有助于CD4+ CAR T细胞在肿瘤治疗中发挥更大作用。
4-1BB CAR
共刺激分子4-1BB是肿瘤坏死因子受体超家族的成员(TNFRSF),与配体结合的4-1BB募集肿瘤坏死因子受体关联因子(TRAF)到4-1BB的胞内结构域形成复合物,通过级联放大作用上调核转录因子NF-κB和JNK通路的活性27,最终促进4-1BB介导的T细胞增殖、存活和T细胞效应因子的表达28。4-1BB还能够诱导细胞端粒酶活性,激活T细胞的活化29。4-1BB通过激活丝裂原活化蛋白激酶(MAPK)通路参与过氧化物酶体增殖物激活受体γ辅激活因子1α(PGC1α)介导的脂质代谢途径,增强T细胞线粒体的功能,维持记忆T细胞的功能30。
4-1BB与CD28是目前CAR胞内结构域中应用最多的共刺激分子,通过小鼠实验发现包含4-1BB胞内信号的CAR T细胞比包含CD28胞内信号的CAR T细胞表达更高的抗凋亡蛋白Bcl-2和Bcl-XL,4-1BB胞内信号有助于增强CAR T细胞的增殖、存活和细胞因子分泌31。4-1BB胞内信号传导还能维持CAR T细胞长效抗肿瘤作用32。研究表明,通过反复抗原刺激CAR T细胞,4-1BB胞内信号可以改善CAR T细胞的耗竭水平13 33,并增加CD8+ 中央记忆T(TCM)细胞的比例,具有中央记忆表型的4-1BB CAR T细胞通过持续分泌IFN-γ来增强抗肿瘤活性34。研究发现4-1BB胞内信号增强CAR T的功能需要依赖于NF-κB和TRAF的调控35,4-1BB胞内信号还促进CAR T细胞的脂肪酸氧化,增强线粒体的氧化呼吸能力36。临床研究表明,4-1BB胞内信号能够维持CAR T细胞在血液肿瘤患者体内的长效抗肿瘤功能,同时引起更少的神经毒性和细胞因子风暴17。目前的研究表明,4-1BB的胞内信号主要作用于CD8+ CAR T细胞,维持CAR T细胞抗肿瘤功能,其对CD4+ CAR T细胞的作用有待进一步的研究。
CD27 CAR
CD27也是肿瘤坏死因子受体超家族的成员,在NK细胞、B细胞以及T细胞上表达37-39。CD27在静止和记忆T细胞中有表达,并在T细胞激活后被上调表达。CD27激活会促进T细胞的增殖和存活31 40,维持记忆T细胞的发育41。CD27刺激既可以诱导效应细胞的扩增和存活,又可以增强记忆细胞的发育,维持T细胞长期的免疫功能42。CD27激活上调T细胞表达抗凋亡蛋白Bcl-XL,抵抗Fas和Fas配体(Fas-L)介导的效应细胞凋亡的影响43,从而促进T细胞存活和记忆细胞的形成44。CD27信号激活可增强周围组织中的T细胞功能45。CD27激活T细胞上调表达IL-2、IFN-γ等效应因子46。与共刺激分子4-1BB一样,CD27刺激会驱动TRAF介导的JNK激活和NF-κB信号通路27。CD27信号还能诱导CD4+ T细胞的分化为Th1细胞,Th1细胞能够抑制肿瘤的生长42。CD27激活通过诱导T细胞上调表达趋化因子CXCL10,促进T细胞的趋化作用和DC细胞的募集47。
尽管共刺激分子CD27对CD4+和CD8+ T细胞的活化起到重要作用,但尚不清楚CD27胞内信号传导对CAR T细胞功能的影响。只有Song等报道,融合了CD27胞内信号区段的CAR,在抗原刺激后能够诱导CAR T细胞Bcl-XL蛋白的表达增加,提高了CAR T细胞的抗凋亡能力,增加了T细胞在体外的扩增48 49。虽然CD27信号激活能上调T细胞的IL-7受体(IL-7R)的表达,也有文献报道通过激活IL-7和IL-7R的信号通路能够显著增强CAR T细胞的抗肿瘤疗效50。但共刺激分子CD27胞内信号对CAR T细胞的抗肿瘤作用尚不清楚,关于融合CD27胞内信号构建的CAR的研究还非常少,CD27胞内信号是否能够有助于CAR T细胞在实体瘤中的应用还有待深入研究。
OX40 CAR
共刺激分子OX40与CD27和4-1BB一样,也是TNFRSF的成员。OX40在活化的T细胞上表达。OX40参与调控T细胞的活化、增殖、分化以及效应因子的表达。研究表明OX40诱导T细胞增殖与4-1BB相似,也是通过TRAF的募集作用。OX40的激活介导TRAF2的活化,诱导NF-κB信号通路途径51,促进了PI3K-AKT途径提高细胞存活和细胞周期,从而诱导抗凋亡基因表达52。激活OX40可以增加肿瘤浸润调节性T细胞(Treg)的糖酵解和脂肪酸合成相关基因53。研究发现,OX40优先在活化的CD4+ T上表达54,缺乏OX40的CD4+ T细胞被激活后无法保持抗凋亡蛋白Bcl-2的高水平表达55,表明OX40对CD4+ T细胞上的作用十分重要。OX40还参与调控CD4+ T细胞的分化为Th1和Th2细胞。与4-1BB 主要作用于CD8+ 记忆T细胞不同,OX40主要作用于CD4+ 记忆T细胞的产生56。
目前,共刺激分子OX40胞内信号更多的是应用在第三代CAR结构中57。体外实验研究发现,OX40胞内信号对CAR T细胞的作用类似于4-1BB胞内信号58。融合OX40胞内信号的三代CAR发现,OX40胞内信号可以促进CAR T细胞产生更多的IFN-γ和IL-17等促炎因子59。联合CD28与OX40胞内信号可增强CAR T细胞抵抗激活诱导的细胞死亡,维持效应细胞的持续抗肿瘤活性60。具有OX40胞内信号的第三代CAR可抑制CAR T细胞因CD28胞内信号诱导的IL-10分泌,但不影响炎性因子分泌和CAR T细胞增殖61。最新研究发现,CAR结构中共表达全长OX40共刺激分子,能够增强了CAR T细胞的增殖并减少T细胞凋亡,维持CAR T细胞毒性,并且降低了耗竭分子的表达,从而增强了CAR T细胞在实体瘤的免疫抑制性肿瘤微环境中的抗肿瘤作用62。OX40胞内信号主要作用于CD4+ CAR T细胞,增强 CAR T细胞的存活63,但OX40胞内信号调控CAR T细胞的具体机制还有待进一步的研究。
总结
CAR的激活是通过胞外抗原识别区段scFv与肿瘤细胞表面抗原的直接结合,进而激活T细胞。CAR活化T细胞的信号强度取决于scFv的亲和力以及CAR分子与靶抗原之间的空间距离。CAR的激活缺乏CD4和CD8与MHC的相互作用的增强信号,所以CAR信号的传导不能完美的模拟TCR信号,致使CAR引起的胞内磷酸化水平与TCR引起的信号强度并不完全相同。共刺激信号可以降低TCR的阈值,促进T细胞的完整活化,而CAR结构中包含的不同共刺激分子胞内信号对CAR信号的传导作用强度也不相同,深入了解不同共刺激分子对CAR T细胞的作用,有助于精准发挥CAR T细胞在不同肿瘤的治疗作用。
CD28和ICOS为CD28超家族成员,促进T细胞的快速增殖,其胞内信号主要介导CAR T细胞的效应功能,其中CD28和ICOS促进有氧糖酵解,并且CD28胞内信号快速诱导CAR T的增殖,对需要大剂量的CAR T细胞的实体瘤治疗或许有益。而TNFR超家族的成员4-1BB、CD27、OX40主要参与记忆T细胞的调控,4-1BB胞内信号主要介导CAR T细胞的脂质代谢和线粒体功能,诱导抗凋亡分子的表达。不同共刺激分子调控不同亚群的T细胞:ICOS主要作用于CD4+ T细胞,诱导Th17细胞分化;4-1BB主要作用于CD8+ T细胞;OX40主要作用于CD4+ T细胞,诱导Th1细胞分化;而CD27可能同样参与了初始CD4+和CD8+ T细胞的应答及扩增阶段。不同共刺激分子胞内信号作用CAR T细胞调控细胞因子的表达也不相同:CD28胞内信号诱导CAR T细胞表达更高的IL-2;而ICOS胞内信号诱导CAR T细胞表达IL-17等炎性细胞因子;OX40胞内信号能够抑制CAR T细胞抗炎因子IL-10的表达;CD27虽然没有发现其诱导CAR T细胞的细胞因子表达,但CD27可以诱导T细胞IL-7R的重新表达,促进T细胞对IL-7的反应性。不同的淋巴细胞亚群、细胞分化水平、代谢途径、以及不同细胞因子的表达均对CAR T细胞的抗肿瘤免疫起到不同的作用。针对血液肿瘤,CAR T细胞能充分接触肿瘤细胞,CAR T细胞在体内的持久性就显得尤为重要。其中,4-1BB激活增强CD8+ CAR T细胞的抗凋亡和维持记忆T细胞功能,是血液瘤治疗中CAR结构胞内共刺激分子很好的选择。对于实体瘤抑制性的免疫微环境,基于肿瘤的异质性和免疫微环境差异,需要探讨选择CAR结构中何种胞内共刺激信号,才能满足CAR T细胞长期存活;维持CAR T细胞的强效的抗肿瘤作用;同时对CD4+ 与CD8+ T细胞都有作用,帮助CAR T细胞突破实体瘤免疫治疗的瓶颈,实现CAR T细胞在肿瘤治疗中的精准应用。
综上所述,通过研究不同共刺激分子对T细胞的调控作用及其胞内信号增强CAR T细胞的机制,有助于在不同肿瘤免疫微环境背景下,CAR T细胞治疗的策略选择。明确适用不同免疫抑制条件下的CAR受体设计和精准应用,进而充分发挥CAR T细胞在肿瘤治疗中的作用潜力,为实现其最大的临床应用价值奠定理论和实验基础。
参考文献
1 Kershaw MH, Westwood JA, Darcy PK. Gene-engineered T cells for cancer therapy. Nature reviews Cancer. 2013;13(8):525-41.
2 Lim WA, June CH. The Principles of Engineering Immune Cells to Treat Cancer. Cell. 2017;168(4):724-40.
3 Brocker T. Chimeric Fv-zeta or Fv-epsilon receptors are not sufficient to induce activation or cytokine production in peripheral T cells. Blood. 2000;96(5):1999-2001.
4 Srivastava S, Riddell SR. Engineering CAR-T cells: Design concepts. Trends in immunology. 2015;36(8):494-502.
5 Restifo NP, Dudley ME, Rosenberg SA. Adoptive immunotherapy for cancer: harnessing the T cell response. Nat Rev Immunol. 2012;12(4):269-81.
6 Fesnak AD, June CH, Levine BL. Engineered T cells: the promise and challenges of cancer immunotherapy. Nature reviews Cancer. 2016;16(9):566-81.
7 Wang Z, Wu Z, Liu Y, et al. New development in CAR-T cell therapy. Journal of hematology & oncology. 2017;10(1):53.
8 Wilcox RA. A three-signal model of T-cell lymphoma pathogenesis. American Journal of Hematology. 2016;91(1):113-22.
9 Esensten JH, Helou YA, Chopra G, et al. CD28 Costimulation: From Mechanism to Therapy. Immunity. 2016;44(5):973-88.
10 Thaker YR, Schneider H, Rudd CE. TCR and CD28 activate the transcription factor NF-kappaB in T-cells via distinct adaptor signaling complexes. Immunology letters. 2015;163(1):113-9.
11 Pegram HJ, Park JH, Brentjens RJ. CD28z CARs and armored CARs. Cancer journal (Sudbury, Mass). 2014;20(2):127-33.
12 Marko AJ, Miller RA, Kelman A, et al. Induction of glucose metabolism in stimulated T lymphocytes is regulated by mitogen-activated protein kinase signaling. PLoS One. 2010;5(11):e15425.
13 Kawalekar OU, O’Connor RS, Fraietta JA, et al. Distinct Signaling of Coreceptors Regulates Specific Metabolism Pathways and Impacts Memory Development in CAR T Cells. Immunity. 2016;44(2):380-90.
14 Klein Geltink RI, O’Sullivan D, Corrado M, et al. Mitochondrial Priming by CD28. Cell. 2017;171(2):385-97.e11.
15 Zheng Y, Collins SL, Lutz MA, et al. A role for mammalian target of rapamycin in regulating T cell activation versus anergy. J Immunol. 2007;178(4):2163-70.
16 Guedan S, Madar A, Casado-Medrano V, et al. Single residue in CD28-costimulated CAR-T cells limits long-term persistence and antitumor durability. The Journal of clinical investigation. 2020;130(6):3087-97.
17 Abramson JS, McGree B, Noyes S, et al. Anti-CD19 CAR T Cells in CNS Diffuse Large-B-Cell Lymphoma. N Engl J Med. 2017;377(8):783-4.
18 Carreno BM, Collins M. The B7 family of ligands and its receptors: new pathways for costimulation and inhibition of immune responses. Annual review of immunology. 2002;20:29-53.
19 Stone EL, Pepper M, Katayama CD, et al. ICOS coreceptor signaling inactivates the transcription factor FOXO1 to promote Tfh cell differentiation. Immunity. 2015;42(2):239-51.
20 Wan Z, Shao X, Ji X, et al. Transmembrane domain-mediated Lck association underlies bystander and costimulatory ICOS signaling. Cellular & molecular immunology. 2020;17(2):143-52.
21 Pulè MA, Straathof KC, Dotti G, et al. A chimeric T cell antigen receptor that augments cytokine release and supports clonal expansion of primary human T cells. Molecular Therapy. 2005;12(5):933-41.
22 Wallin JJ, Liang L, Bakardjiev A, et al. Enhancement of CD8+ T cell responses by ICOS/B7h costimulation. Journal of immunology. 2001;167(1):132-9.
23 Chen L, Flies DB. Molecular mechanisms of T cell co-stimulation and co-inhibition. Nature reviews Immunology. 2013;13(4):227-42.
24 Zeng H, Cohen S, Guy C, et al. mTORC1 and mTORC2 Kinase Signaling and Glucose Metabolism Drive Follicular Helper T Cell Differentiation. Immunity. 2016;45(3):540-54.
25 Guedan S, Posey AD, Jr., Shaw C, et al. Enhancing CAR T cell persistence through ICOS and 4-1BB costimulation. JCI insight. 2018;3(1).
26 Guedan S, Chen X, Madar A, et al. ICOS-based chimeric antigen receptors program bipolar TH17/TH1 cells. Blood. 2014;124(7):1070-80.
27 McPherson AJ, Snell LM, Mak TW, et al. Opposing roles for TRAF1 in the alternative versus classical NF-κB pathway in T cells. The Journal of biological chemistry. 2012;287(27):23010-9.
28 Li G, Boucher JC, Kotani H, et al. 4-1BB enhancement of CAR T function requires NF-κB and TRAFs. JCI insight. 2018;3(18).
29 Ahmad HT, Mansooreh J, Fereshteh M, et al. Changes in lymphocytes’ telomerase activity by 4-1BB costimulation. Journal of cancer research and therapeutics. 2014;10(4):998-1003.
30 Menk AV, Scharping NE, Rivadeneira DB, et al. 4-1BB costimulation induces T cell mitochondrial function and biogenesis enabling cancer immunotherapeutic responses. The Journal of experimental medicine. 2018;215(4):1091-100.
31 Croft M. Costimulation of T cells by OX40, 4-1BB, and CD27. Cytokine & Growth Factor Reviews. 2003;14(3-4):265-73.
32 Imai C, Mihara K, Andreansky M, et al. Chimeric receptors with 4-1BB signaling capacity provoke potent cytotoxicity against acute lymphoblastic leukemia. Leukemia. 2004;18(4):676-84.
33 Sacchetti B, Botticelli A, Pierelli L, et al. CAR-T with License to Kill Solid Tumors in Search of a Winning Strategy. Int J Mol Sci. 2019;20(8).
34 Labanieh L, Majzner RG, Mackall CL. Programming CAR-T cells to kill cancer. Nat Biomed Eng. 2018;2(6):377-91.
35 Zapata JM, Perez-Chacon G, Carr-Baena P, et al. CD137 (4-1BB) Signalosome: Complexity Is a Matter of TRAFs. Frontiers in immunology. 2018;9:2618.
36 Golubovskaya V, Wu L. Different Subsets of T Cells, Memory, Effector Functions, and CAR-T Immunotherapy. Cancers. 2016;8(3).
37 Grant EJ, Nüssing S, Sant S, et al. The role of CD27 in anti-viral T-cell immunity. Current Opinion in Virology. 2017;22:77-88.
38 Phan TG, Tangye SG. Memory B cells: total recall. Current opinion in immunology. 2017;45:132-40.
39 Muntasell A, Ochoa MC, Cordeiro L, et al. Targeting NK-cell checkpoints for cancer immunotherapy. Current opinion in immunology. 2017;45:73-81.
40 Burris HA, Infante JR, Ansell SM, et al. Safety and Activity of Varlilumab, a Novel and First-in-Class Agonist Anti-CD27 Antibody, in Patients With Advanced Solid Tumors. Journal of clinical oncology : official journal of the American Society of Clinical Oncology. 2017;35(18):2028-36.
41 Ramakrishna V, Sundarapandiyan K, Zhao B, et al. Characterization of the human T cell response to in vitro CD27 costimulation with varlilumab. Journal for immunotherapy of cancer. 2015;3:37.
42 Hendriks J, Gravestein LA, Tesselaar K, et al. CD27 is required for generation and long-term maintenance of T cell immunity. Nature immunology. 2000;1(5):433-40.
43 Dolfi DV, Boesteanu AC, Petrovas C, et al. Late signals from CD27 prevent Fas-dependent apoptosis of primary CD8+ T cells. J Immunol. 2008;180(5):2912-21.
44 Arens R, Schepers K, Nolte MA, et al. Tumor rejection induced by CD70-mediated quantitative and qualitative effects on effector CD8+ T cell formation. The Journal of experimental medicine. 2004;199(11):1595-605.
45 Hendriks J, Xiao Y, Borst J. CD27 promotes survival of activated T cells and complements CD28 in generation and establishment of the effector T cell pool. The Journal of experimental medicine. 2003;198(9):1369-80.
46 Takeda K, Oshima H, Hayakawa Y, et al. CD27-mediated activation of murine NK cells. J Immunol. 2000;164(4):1741-5.
47 Peperzak V, Veraar EA, Xiao Y, et al. CD8+ T cells produce the chemokine CXCL10 in response to CD27/CD70 costimulation to promote generation of the CD8+ effector T cell pool. J Immunol. 2013;191(6):3025-36.
48 Song DG, Ye Q, Poussin M, et al. CD27 costimulation augments the survival and antitumor activity of redirected human T cells in vivo. Blood. 2012;119(3):696-706.
49 Song D-G, Powell DJ. Pro-survival signaling via CD27 costimulation drives effective CAR T-cell therapy. OncoImmunology. 2014;1(4):547-9.
50 Dong H, Franklin NA, Roberts DJ, et al. CD27 stimulation promotes the frequency of IL-7 receptor-expressing memory precursors and prevents IL-12-mediated loss of CD8(+) T cell memory in the absence of CD4(+) T cell help. J Immunol. 2012;188(8):3829-38.
51 Croft M. The role of TNF superfamily members in T-cell function and diseases. Nature reviews Immunology. 2009;9(4):271-85.
52 So T, Choi H, Croft M. OX40 complexes with phosphoinositide 3-kinase and protein kinase B (PKB) to augment TCR-dependent PKB signaling. J Immunol. 2011;186(6):3547-55.
53 Pacella I, Procaccini C, Focaccetti C, et al. Fatty acid metabolism complements glycolysis in the selective regulatory T cell expansion during tumor growth. Proceedings of the National Academy of Sciences of the United States of America. 2018;115(28):E6546-e55.
54 Taraban VY, Rowley TF, O’Brien L, et al. Expression and costimulatory effects of the TNF receptor superfamily members CD134 (OX40) and CD137 (4-1BB), and their role in the generation of anti-tumor immune responses. European journal of immunology. 2002;32(12):3617-27.
55 Sun G, Sun X, Li W, et al. Critical role of OX40 in the expansion and survival of CD4 T-cell-derived double-negative T cells. Cell death & disease. 2018;9(6):616.
56 Salek-Ardakani S, Song J, Halteman BS, et al. OX40 (CD134) controls memory T helper 2 cells that drive lung inflammation. The Journal of experimental medicine. 2003;198(2):315-24.
57 Hillerdal V, Ramachandran M, Leja J, et al. Systemic treatment with CAR-engineered T cells against PSCA delays subcutaneous tumor growth and prolongs survival of mice. BMC cancer. 2014;14:30.
58 Finney HM, Akbar AN, Lawson AD. Activation of resting human primary T cells with chimeric receptors: costimulation from CD28, inducible costimulator, CD134, and CD137 in series with signals from the TCR zeta chain. J Immunol. 2004;172(1):104-13.
59 Wilkie S, Picco G, Foster J, et al. Retargeting of human T cells to tumor-associated MUC1: the evolution of a chimeric antigen receptor. J Immunol. 2008;180(7):4901-9.
60 Hombach AA, Chmielewski M, Rappl G, et al. Adoptive immunotherapy with redirected T cells produces CCR7- cells that are trapped in the periphery and benefit from combined CD28-OX40 costimulation. Human gene therapy. 2013;24(3):259-69.
61 Hombach AA, Heiders J, Foppe M, et al. OX40 costimulation by a chimeric antigen receptor abrogates CD28 and IL-2 induced IL-10 secretion by redirected CD4(+) T cells. Oncoimmunology. 2012;1(4):458-66.
62 Zhang H, Li F, Cao J, et al. A chimeric antigen receptor with antigen-independent OX40 signaling mediates potent antitumor activity. Sci Transl Med. 2021;13(578).
63 Hombach AA, Abken H. Costimulation by chimeric antigen receptors revisited the T cell antitumor response benefits from combined CD28-OX40 signalling. International journal of cancer. 2011;129(12):2935-44.